-
Lithium-ion batteries – electrolytes – solid & semi-solid
-
Multi-doped LLZO solid electrolyte
Li6.75La2.75Ca0.25Zr1.50Ta0.50O12
was prepared, which exhibits a very high ion conductivity of 4.2 × 10-3 S/cm.
As shown in the Figure below, the above electrolyte (503) predominantly exhibits a cubic crystal structure (calculation: 507),
while the comparative undoped
LLZO material (501) predominantly exhibits a tetragonal crystal structure (calculation: 505).
This material was prepared by mixing lithium hydroxide monohydrate, lanthanum oxide,
zirconium (IV) oxide, calcium carbonate and tantalum (V) oxide, followed by sintering
(900°C, ≈12 h, MgO or Pt crucible). This material was milled in isopropanol, and dried (55°C).
The resulting material was mixed with isopropanol, toluene and Z3 menhaden fish oil (dispersant), milled,
and mixed with polyvinyl butyral (binder) and benzyl butyl phthalate (plasticizer).
The slurry was coated (doctor-blading) on a Mylar film, dried (55°C), hot pressed (93°C,
optionally including lamination of porous / dense LLZO layers),
and sintered (900-1,200°C, 1 min to 6 h).
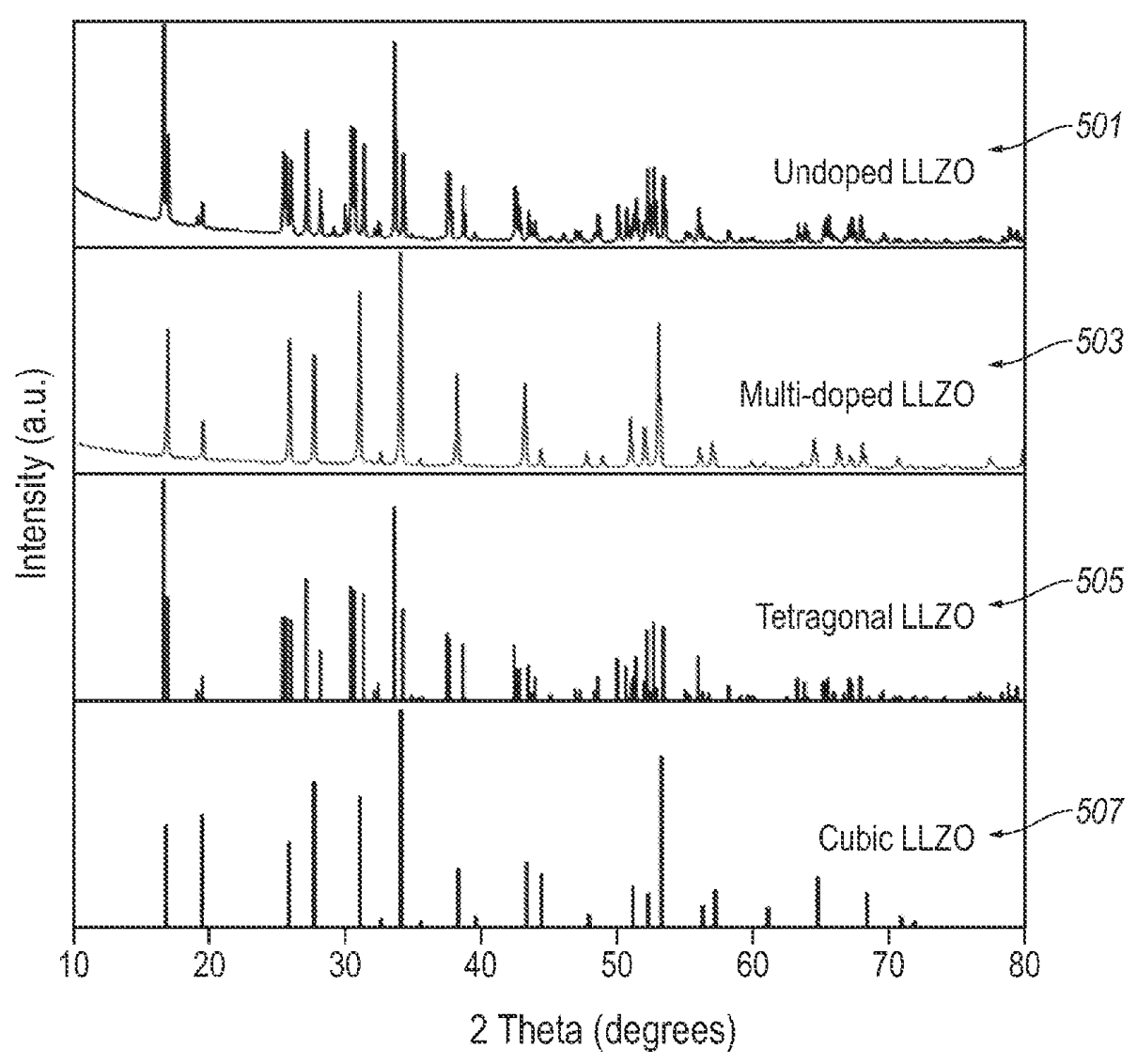
This work illustrates how multi-element doping of LLZO allows for selective formation of the cubic
crystalline phase (which exhibits superior ion conductivity as compared to the tetragonal phase).
This work is applicable to porous-dense electrolyte layers covered in prior patent applications by ION Storage Systems
and also to other electrolyte architectures (sintered LLZO mono-layers and composite layers based on oxide particles / polymers).
-
The premium version includes another two patent discussions, plus an Excel list with 50-100 commercially relevant recent patent families.
-
Lithium-ion batteries – positive electrode
-
A hydroxide precursor was synthesized by mixing NiSO4 · 6 H2O and
MnSO4 · H2O (40 : 60 molar ratio). NaOH and NH4OH were added, and the mixture was stirred (45°C, under nitrogen),
followed by washing and drying to obtain Ni0.4Mn0.6(OH)2.
This material was heat-treated (550°C, 5 h), followed by mixing with LiOH, LiF and MgF2
(1.25 / 0.033 / 0.017 equivalents with respect to Mn + Ni) and a heat treatment (900°C, 8 h).
An SEM image of the resulting active material is shown below. The material exhibits
a BET specific surface area of 1.48 m2/g. In half-cells, the material exhibits a 0.1 C discharge
capacity of 224.2 mAh/g, a first cycle efficiency of 86.4%, and a capacity retention after 50 cycles of 93.5% (1 C charge / discharge),
as compared to 205.1 mAh/g, 85.0%, 91.8% for a comparative material that was not treated with LiF / MgF2, respectively.
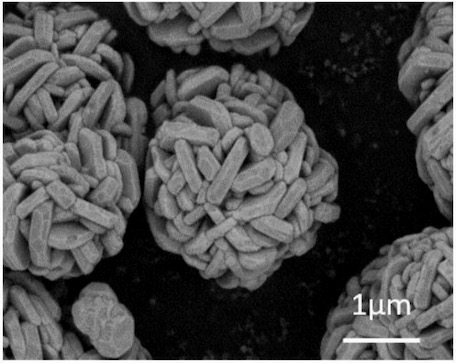
This work illustrates how a surface treatment with LiF / MgF2 leads to improved electrochemical characteristics in LRLO
(lithium-rich layered oxide) active materials and the ability to tune the porous crystallite morphology.
-
The premium version includes another two patent discussions, plus an Excel list with 50-100 commercially relevant recent patent families.
-
Lithium-ion batteries – negative electrode (excluding Li metal electrodes)
-
Semiconductor-grade silicon (≥10 μm), diethylene glycol, and N-allyl-(2-ethylxanthate)propylamide (5 mass%) were ground (2,400-3,000 rpm),
to produce nano-silicon with a grain size of 15-50 nm.
Flaky natural graphite (10 mass%) was added to this mixture, which resulted in a silicon-carbon composite through orderly stacking
of Si particles on the graphite substrate.
This silicon-carbon composite was subsequently mixed with Li22Si5 / Li2O (in total 1 mass%), followed by
co-granulation and a heat treatment (550°C, 8 h, nitrogen / hydrogen gas mixture) to obtain a pre-lithiated Si-carbon
composite material (see SEM image below).
In half-cells, the material exhibits a reversible capacity of 1,700 mAh/g along with a first cycle efficiency of 89.6% and a capacity
retention after 100 cycles of 93.8% (0.1 C charge / discharge), as compared to 1,595 mAh/g, 84.5% and 63.5% for a comparative
material that was not treated with Li22Si5, respectively.
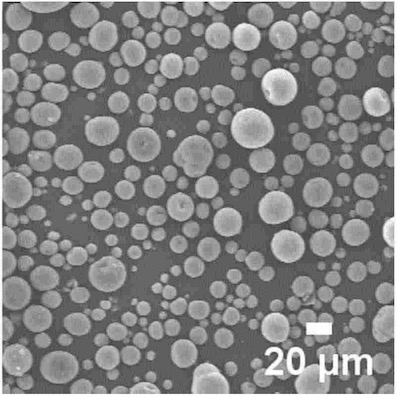
It is claimed that nano-silicon with 15-50 nm grain size can be made upon grinding with diethylene glycol and
N-allyl-(2-ethylxanthate)propylamide, which is a very small grain size as compared to other 'top-down' grinding approaches.
It would be interesting
to know more about the particle size distribution and whether the presence of Si oversize particles was successfully avoided.
Furthermore, this work illustrates how co-granulation in combination with 1 mass%
of Li22Si5 / Li2O results in a highly beneficial effect on electrochemical performance.
The exact Li22Si5 / Li2O ratio was not disclosed.
-
The premium version includes another two patent discussions, plus an Excel list with 50-100 commercially relevant recent patent families.
-
Fuel cells (PEMFC / SOFC / PAFC / AEMFC) – electrochemically active materials
-
Gas diffusion layers (GDL) were prepared based on an electrically conductive non-woven (thickness prior to compressing: 145 μm, 60 g/m2)
coated with a microporous layer (MPL). The MPL exhibits a gradient
across the XY-plane upon coating 4 strips with 4 different aqueous MPL slurries that primarily vary in their plastic pore former
content (0-0.4 mass%) and carbon type (no specifics were identified). After coating, GDLs were dried (160°C) and a heat-treated (400°C).
MPL loading is between 15 and 22 g/m2.
This GDL exhibits a Gurley gas permeability gradient (strip 1: 24.1 s, strip 2: 6.8 s, strip 3: 1.0 s, strip 4: 0.6 s).
It is argued that these gradient characteristics result in a more favorable gas transport with respect to the catalyst layers
in combination with favorable compressibility and electrical conductivity.
This work illustrates how the combination of different carbons and pore former concentrations in MPL slurries allows
for tuning porosity across the XY-plane of GDLs, while also satisfying compressibility and electrical conductivity
requirements.
-
The premium version includes another two patent discussions, plus an Excel list with 50-100 commercially relevant recent patent families.
-
Triweekly patent lists for other categories (Excel files are included for premium users)
-
- Lithium metal containing batteries (excluding Li-S, Li-Air): XLSX
-
- Lithium-ion batteries – electrolytes – liquid: XLSX
-
- Lithium-ion batteries – separators: XLSX
-
- Lithium-sulfur batteries: XLSX
-
- Metal-air batteries: XLSX
-
- Na-ion batteries: XLSX
-
Prior patent updates
-
2024-04-16
-
2024-03-26
-
2024-03-05
-
2024-02-13
-
2024-01-23
|