-
Assessment of Companies
-
Author comments are displayed in maroon.
-
Contemporary Amperex Technology (CATL) – China
-
Organization profile
Contemporary Amperex Technology Limited (CATL, https://www.catl.com/en/)
is the world's
largest Li-ion battery producer
.
CATL was founded in 2011 in Ningde,
China. In 2017, CATL has completed a split from its parent company ATL/TDK.
A commercial sodium-ion battery was launched in 2021.
With BRUNP Recycling (subsidiary)
, and Dynanonic
(publicly traded, >60% floating stock) CATL jointly develops positive electrode
active materials. CATL recently launched LMFP-based M3P liquid electrolyte Li-ion batteries
along with Qiling packs ('cell-to-pack' architecture).
Unique capability: supramolecular ionic liquid / polymer / lithium salt electrolytes with very favorable ion conductivity (up to 2.4 × 10-3 S/cm) and high boiling point (>438 °C), along with corresponding cells with lithium metal negative electrodes.
Leap of faith: the toxicity of triphenylene-containing electrolytes will be acceptable.
Comment: this work could finally enable the operation of lithium metal negative electrodes at room temperature and below
with favorable fast charge /
discharge characteristics (along with favorable energy density), without employing scarce elements in the electrolyte (aside from lithium).
The use
of triphenylene-containing ionic liquids requires sufficiently strict protocols during manufacturing, operation and recycling to avoid PAH
(polycyclic aromatic hydrocarbon) contamination.
-
Possible material / cell / process characteristics (estimate based on public information)
-
Electrolyte: supramolecular ionic liquids with triphenylene cores and (optionally fluorinated) ethylene oxide oligomers /
lithium sulfonate groups (Figure 71, top) exhibit an ion conductivity of up to 6.5 × 10-3 S/cm (R1 / R2 groups on the left).
This ionic liquid was combined with (PEO / PVDF) and LiTFSI (10-80 : 100 : 5-40 by mass, 100 corresponds to combined mass PEO /
PVDF) to form electrolyte films (25 μm thickness) with an ion conductivity of 2.4 × 10-3 S/cm. Boiling point: >438 °C.
-
Negative electrode: lithium metal. Although Li metal processing patents have been filed by CATL, employment of an ‘anode-free’ cell design is also a possibility.
-
Positive electrode: NMC9½½ or NMC811 / conductive carbon / PVDF (96 : 2 : 2 by mass),
see ‘Lithium-ion Battery High-energy Cathode Innovation & Patent Review’.
-
Design: stacked multilayer, prismatic cells.
-
Process:
1) |
Mix electrolyte components. |
2) |
Hot pressing (1-20 MPa, 50-100 °C) to produce electrolyte film. |
3) |
Vacuum annealing (60-80 °C, 1-8 h). |
4) |
Electrode and electrolyte layers were laminated through cold-pressing (250 MPa, 25 °C, 2 min) to obtain 10-layer cells. |
5) |
Encapsulation inside prismatic or pouch cell housing. |
-
News reports & press releases
In April 2023, CATL unveiled its
500 Wh/kg ‘condensed battery’ for electric aircrafts and EVs
(additional article).
The limited technical details that were disclosed are consistent with the description of supramolecular ionic
liquid-based Li metal batteries described in this chapter.
In December 2022,
it was reported that companies CATL and CALB have filed lawsuits with respect to each other related to Li-ion battery intellectual
property (not related to solid-state batteries).
In August 2022, it was reported that CATL plans to introduce ‘condensed batteries’ in 2023, which according to some experts provides favorable safety,
reliability and cycle life by achieving a ‘superfluid state, superconducting state, stability, and superior conduction’.
In January 2022, it was reported that CATL expects that 1st generation solid-state batteries with roughly the same energy density as
current Li-ion batteries will capture about 1% market share by 2030, while 2nd generation solid-state Li-ion batteries with new positive /
negative electrode active materials are expected to emerge after 2030
(additional report).
-
General patent portfolio characteristics
14 new patent families related to semi-solid or solid-state Li-ion batteries have been published since 2021.
-
Examples from the patent portfolio
Level 1) Electrolyte & electrode patents, level 2) cell patents
-
SUPRAMOLECULAR IONIC LIQUIDS, SOLID STATE ELECTROLYTE MEMBRANE, SOLID STATE LITHIUM METAL BATTERY, AND DEVICE
(published in 2022):
Semi-solid electrolyte layer (25 μm): supramolecular ionic liquids with triphenylene cores and
(optionally fluorinated) ethylene oxide oligomers and lithium sulfonate groups (Figure 71) exhibit an ion conductivity
of 6.5 × 10-3 S/cm (R1 / R2 groups on the left). This ionic liquid was combined with (PEO / PVDF) and LiTFSI (10-80 : 100 : 5-40 by mass,
100 corresponds to combined mass PEO / PVDF) to form electrolyte films (25 μm thickness) with an ion conductivity of 2.4 × 10-3 S/cm.
Negative electrode: lithium metal.
Positive electrode: NMC811 / conductive carbon / PVDF (96 : 2 : 2 by mass).
Small-angle X-ray scattering (SAXS) measurements exhibit a channel size of about 17 nm between π-stacked triphenylene units through which Li-ions can diffuse.
Electrolyte layers were produced through hot pressing (1-20 MPa, 50-100 °C), followed by vacuum annealing (60-80 °C, 1-8 h). Electrode and electrolyte layers
were laminated through cold-pressing (250 MPa, 25 °C, 2 min) to obtain 10-layer cells.
In cycling tests (0.5 C charge / discharge), cells that contain triphenylene molecules with R1 / R2 groups shown on the top left of Figure 71 exhibit
409 cycles (prior to reaching 80% remaining capacity cutoff), while cells that contain triphenylene molecules with R1 / R2 groups shown on the top right
of Figure 71 exhibit 1,002 cycles (ion conductivity of molecule: 4.4 × 10-3 S/cm, ion conductivity of PEO / PVDF / LiTFSI-containing
film: 1.8 × 10-3 S/cm).
Figure 71: top – two triphenylene derivatives with (optionally fluorinated) ethylene oxide / lithium sulfonate groups. The derivative on the
left exhibits particularly favorable ion conductivity (6.5 × 10-3 S/cm), while the derivative on the right exhibits particularly favorable
cycling stability, bottom – synthesis procedure, OR3 = R1, OR4 = R2 (CATL)
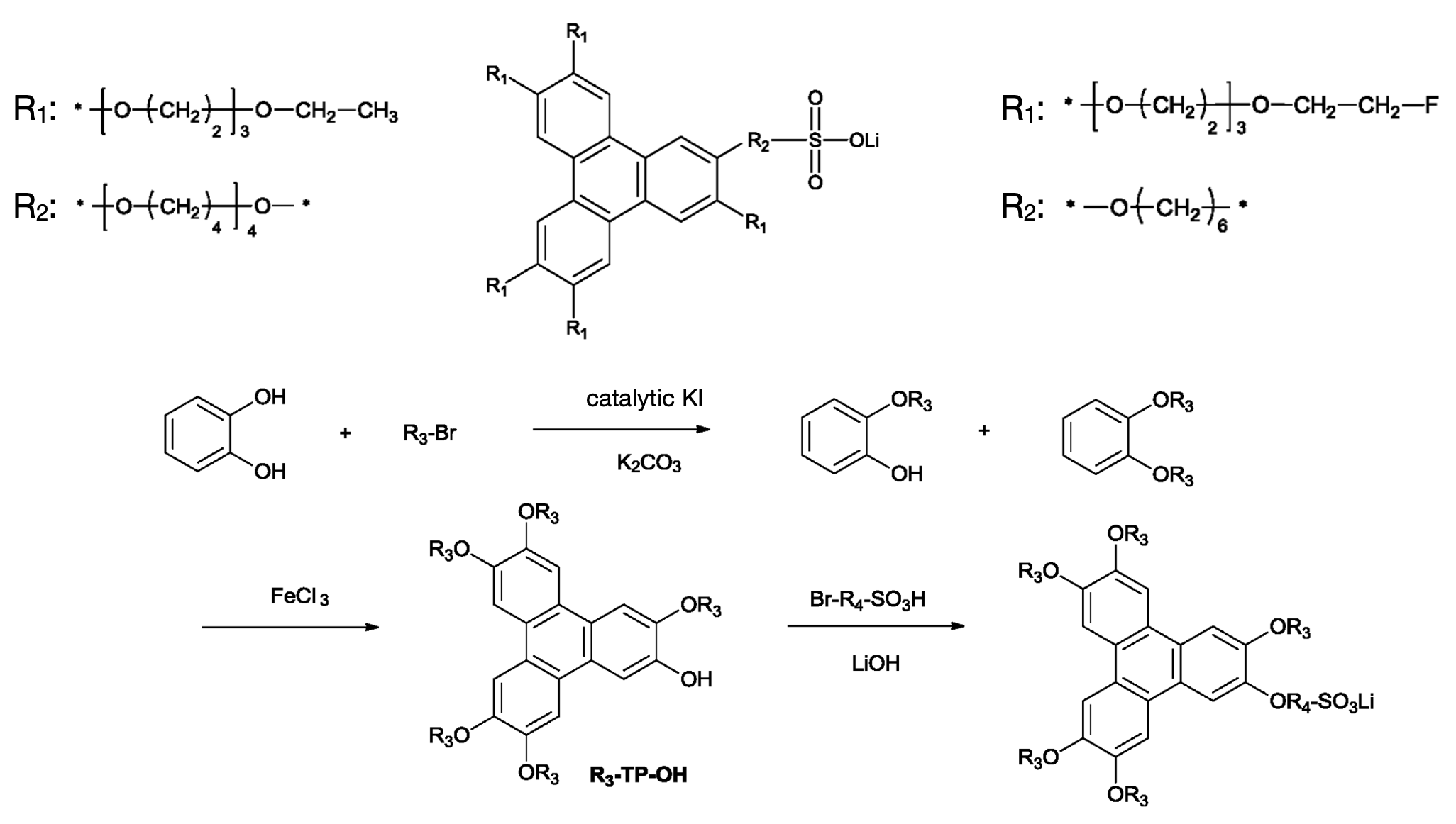
This work illustrates how very favorable ion conductivity and cycling characteristics can be achieved upon incorporating a polycyclic aromatic hydrocarbon
(triphenylene) that undergoes π-stacking interactions to form nano-columns. Supramolecular aggregates presumably are substantially immobile in the electrolyte
layer, while providing functional PEG and lithium sulfonate edge groups that promote Li-ion diffusion.
The resulting electrolyte layer apparently prevents short circuits due to lithium dendrite formation by exhibiting sufficient mechanical resistance in the
absence of cracks through which lithium dendrites could grow (self-healing character of supramolecular interactions).
The SEI layer that likely forms between the electrolyte and the lithium metal layer presumably is sufficiently supported by the electrolyte layer as not
to crack during cycling (avoidance of excess SEI formation).
As triphenylene (without further functional groups) exhibits a boiling point of 438 °C, the safety characteristics of this electrolyte system could be more
favorable as compared to plasticizers that exhibit a lower boiling point.
A potential disadvantage relates to the toxicity of polycyclic aromatic hydrocarbons (PAH).
Presumably, a coating on NMC811 is deployed that prevents decomposition of ethylene oxide groups at high voltages.
The synthesis procedure shown in Figure 71 (bottom) suggests that large scale chemical synthesis should be possible without overly expensive reactants or
process conditions.
-
Solid-state electrolyte membrane, solid-state battery and device (published in 2022):
Solid electrolyte layer (20-30 μm): Li6PS5 / binder (80 : 20 by mass, binder type: probably SBR,
screened with specific mesh number, 99 mass% of particles exhibit a particle size of 5-20 μm).
Ion conductivity: 1.2 × 10-3 S/cm.
Positive electrode: NMC / Li6PS5 / carbon black (Super P, IMERYS Graphite & Carbon) / SBR binder
(70 : 24 : 3 : 3 mass ratio), on aluminum foil.
Negative electrode: lithium foil, on copper foil.
Cells with an electrolyte layer thickness of 30 μm (303 Wh/kg energy density) exhibit 165 cycles
until 50% capacity is reached (0.1 C charge / discharge). Cells with an electrolyte layer thickness
of 20 μm (310 Wh/kg energy density) exhibit 116 cycles under the same conditions. Cells without SBR
binder with an electrolyte layer thickness of 30 μm exhibit 9 cycles under the same conditions.
Electrolyte and electrodes were prepared, in case of the electrolyte layer through ball milling (in toluene),
in case of the positive electrode through slurry mixing (in NMP). These layers were laminated with the negative
electrode (pressing at 300 MPa).
It is argued that a solid electrolyte layer breaking strength of >50 MPa supports favorable electrochemical performance.
This work illustrates the importance of using a mechanically flexible binder in combination with sulfide.
-
Included in full version: further patent discussion.
Level 3A) Module / form factor / packaging patents; level 3B) application patents; level 3C) reliability patents
|