-
Lithium-ion batteries – electrolytes – solid & semi-solid
-
Two nickel-mesh substrates (8 cm × 11 cm, areal density of 0.13 g/cm2,
>99% pure Ni, Si <0.35%, Fe <0.40%, 200 grade, Belleville Wire Cloth Company) were cleaned in isopropanol via ultrasonication (5 min).
The first substrate was placed on a nickel
plate (thickness: 1.56 mm), and undoped LLZO powder (e.g. Li7La3Zr2O12) was spread evenly across it.
The second substrate was placed on top of the oxide electrolyte precursor powder bed,
followed by another nickel plate. The stack was heated (1,100°C, 2 h, increase at 3°C/min, 0.4% H2 in Ar, pO2
<10-23 atm).
An XPS (X-ray Photoelectron Spectroscopy) analysis after the sintering procedure reveals a uniform coating of the nickel plate
with Li2CO3,
with traces of Li2O at the surface.
A coated setter body with a nickel plate substrate was placed in a furnace with a green body (precursor)
bilayer tape based on doped LLZO. The tape included a porous layer (40 μm thick) with a pore forming agent and a dense layer
with excess lithium (15% above stoichiometric from Li2CO3). The porous layer was placed in contact with the setter body,
and a nickel-mesh setter body was placed on top. The stack was heated (increase at 0.4°C/min to 580°C in O2, hold for 30 min) to burn
out the binder and pore forming agent, followed a heat-treatment under argon (30 min, 580°C) and under 0.4% H2 in Ar (30 min, 580°C,
followed by increase at 1°C/min to 725°C, hold for 1 h, followed by increase at 3°C/min, hold for 2 h).
As shown in the Figure, the setter bodies were recovered and reused in eleven sintering processes.
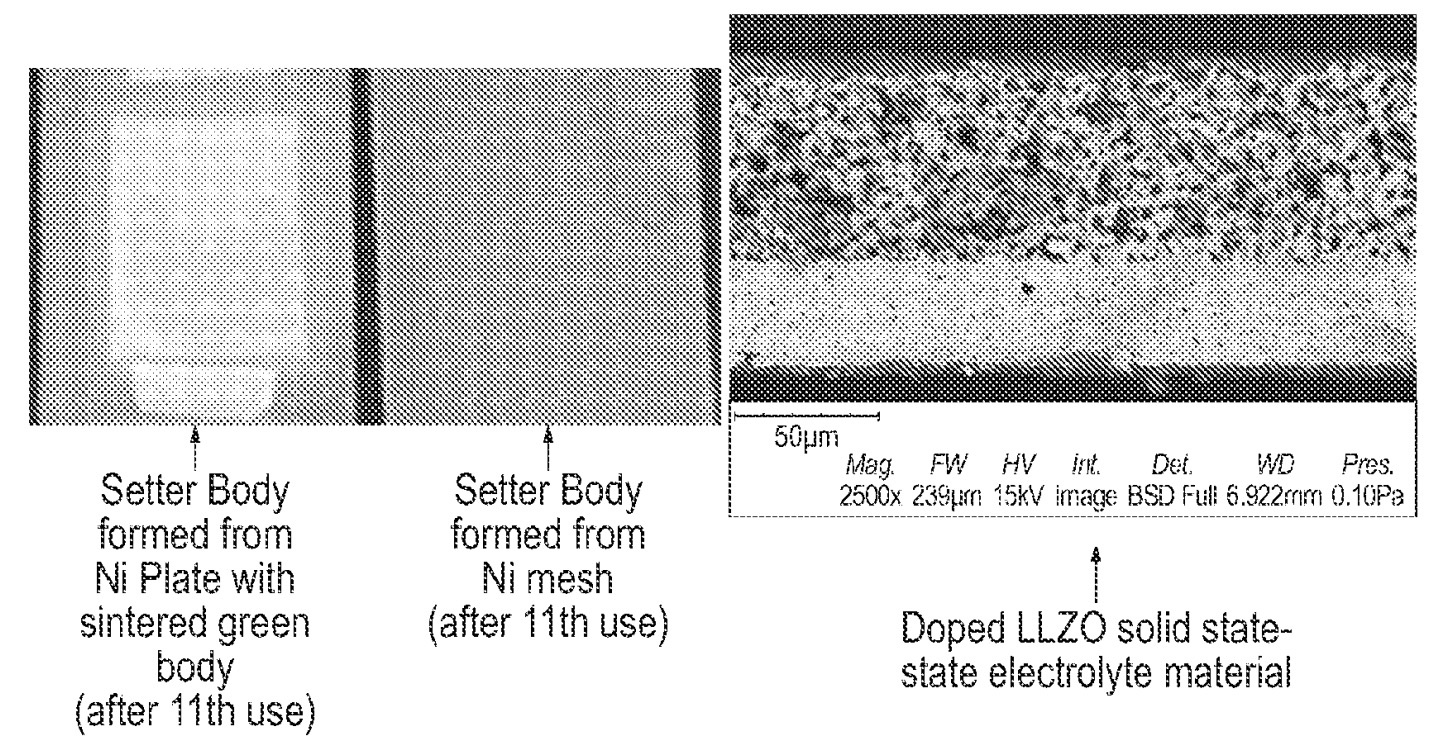
This work illustrates how Ion Storage Systems is optimizing the process efficiency for its bi-layer oxide electrolyte layer, although
heating to a very high temperature of 1,100°C apparently remains necessary to assure favorable fast charge rates with lithium metal electrodes.
This approach of optimizing process efficiency without sacrificing performance is consistent with a go-to-market approach that first
targets high-value niche (probably military or drone) applications.
-
The premium version includes another two patent discussions, plus an Excel list with 50-100 commercially relevant recent patent families.
-
Lithium-ion batteries – negative electrode (excluding Li metal electrodes)
-
Porous carbon underwent a first chemical vapor deposition with simultaneous
monosilane and lithium amide gases to form a silicon-carbon precursor (400-800°C, ≈50 min, ≈30 kPa, presence of inert gas). This was
followed by a second deposition with acetylene to produce the final silicon-carbon
material (400-800°C, ≈10 h, presence of inert gas). The resulting active material exhibits a carbon / Si / Li content
of ≈ 50 : 46 : 4 by mass.
The Figure shows the apparatus with a gas generator (1), mixer (2), and reactor (3).
The mixer features gas inlets (101, 102) and a deposition gas outlet (103). Phenyl lithium and lithium vapor are mentioned as
alternative Li sources.
Negative electrode slurry composition: CMC (carboxymethyl cellulose) : PAA (polyacrylic acid) : SBR (styrene-butadiene rubber) :
SP (Super P carbon black) : CNTs (carbon nanotubes) : silicon-carbon material : graphite = 0.5 : 3.5 : 0.5 : 0.1 : 0.1 : 10 : 85.3 by mass ratio.
In half-cell tests, the co-deposited material exhibits a first cycle efficiency of 120.4%
at 0.8 V versus 82.1% for a comparative material without lithium.
Cycling stability reached 932 cycles (60% capacity cutoff, 0.5 C charge / discharge), as compared to 132 cycles for the
comparative material.
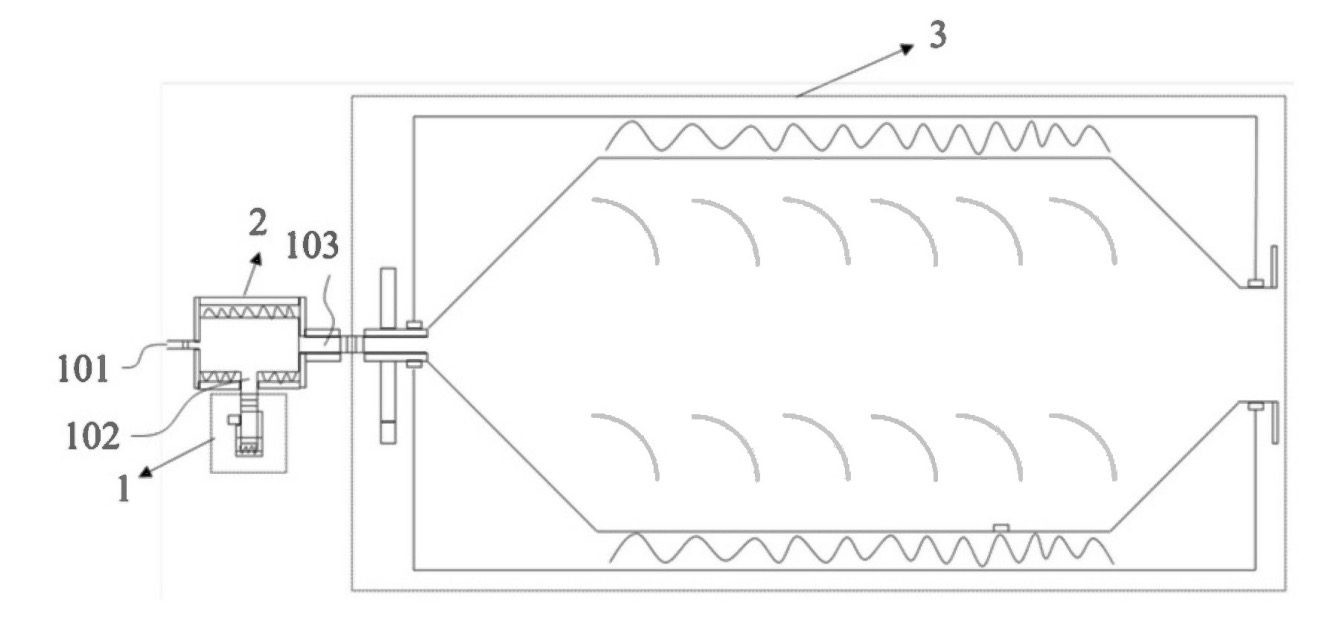
This work illustrates that lithiation of Si-carbon composites is feasible simultaneously to Si CVD deposition.
More detailed investigations might be necessary to confirm that the lithiated material can be processed in water-based electrode slurry
formulations. An interesting question is also whether such lithiated Si-carbon composite materials are particularly suitable for
dry electrode formation processes.
-
The premium version includes another two patent discussions, plus an Excel list with 50-100 commercially relevant recent patent families.
-
Lithium-ion batteries – positive electrode
-
MnPO4 powder, FePO4 powder,
Li2CO3 powder, TiO2 powder (0.5 mass% of the total lithium metal phosphate mass),
and glucose (10 mass% of the total lithium metal phosphate mass) were ball-milled in distilled water
(200 tpm, 60 min, zirconia balls), followed by spray-drying and calcination (650°C, 4 h, nitrogen).
The mixture was ball-milled at 200 rpm for 60 min using zirconia balls to form a slurry.
After removing the zirconia balls, the slurry was spray-dried to obtain a powder
mixture.
These particles
were pulverized and classified using a jet mill to obtain LMFP (lithium manganese iron phosphate)
particles with a median diameter of 1.5 μm.
As shown in the Figure, the charge-discharge profile exhibits characteristic plateaus,
with an iron-derived plateau at ≈3.5V and a manganese-derived plateau at ≈4.1V.
The crystallite size in the (020) plane direction, measured by X-ray diffraction and
calculated using the Scherrer equation, is 215 nm. In half-cells this material exhibits an
initial discharge capacity of 152.2 mAh/g and a first cycle efficiency of 97.9%.
실시예 1: Example 1
비교예 1: Comparative Example 1 (LiMn0.6Fe0.4PO4 without Ti)
비교예 2: Comparative Example 2 (LiMn0.65Fe0.35PO4 without doping)
비교예 3: Comparative Example 3 (LiMn0.7Fe0.3PO4 without doping)
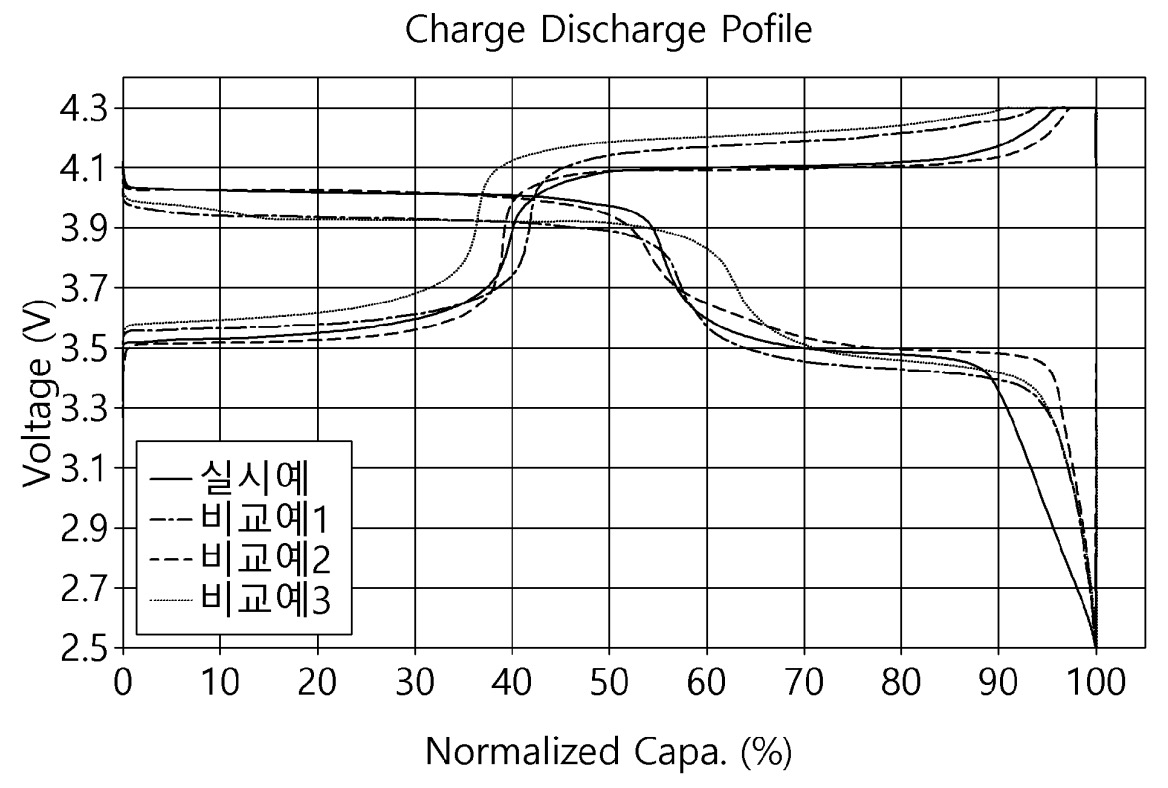
This work illustrates that SK On engages in the development of Ti-doped / carbon-coated LMFP active materials.
-
The premium version includes another two patent discussions, plus an Excel list with 50-100 commercially relevant recent patent families.
-
Fuel cells (PEMFC / SOFC / PAFC / AEMFC) – electrochemically active materials
-
A near-surface phosphorus-doped platinum-carbon fuel cell catalyst was prepared.
Chloroplatinic acid, EC300-JD carbon black, and sodium dihydrogen phosphate were
mixed in water. After ultrasonic dispersion and stirring (14 h), the mixture was
rotary-evaporated to remove water. The resulting P-Pt/C catalyst precursor was
heat-treated in a reducing atmosphere (5% H2 in Ar) at 500°C for 2 h.
The resulting platinum-carbon catalyst contains 20-62 mass% Pt and 0.5-7 mass%
non-metallic P, with the remainder being carbon support. The catalyst exhibits
platinum nanoparticles with an average diameter of 2.6 nm distributed on the
carbon support surface (see Figure), with phosphorus atoms doped in the near-surface region of
the platinum nanoparticles, located 1-2 atomic layers below the surface.
The catalyst exhibits superior
electrochemical stability compared to non-doped or nitrogen-doped platinum-carbon
catalysts. This enhanced stability results from phosphorus's relatively weak
electronegativity, which allows its electronic structure to modulate the oxygen
reduction performance of the platinum-carbon catalyst without significantly
altering the platinum nanoparticle crystal structure.
The phosphorus-doped catalyst exhibits an initial ORR (oxygen reduction reaction) half-wave potential of 0.94 V
and 3.5 mV loss after 30,000 cycles (mass activity loss: 8.8%, cycling 0.6-1.0 V). In comparison, a catalyst prepared without
phosphorus doping exhibits significantly worse performance with an initial ORR
half-wave potential of 0.90 V, a half-wave potential decay of 7.3 mV, and
a mass activity loss of 27.3%.
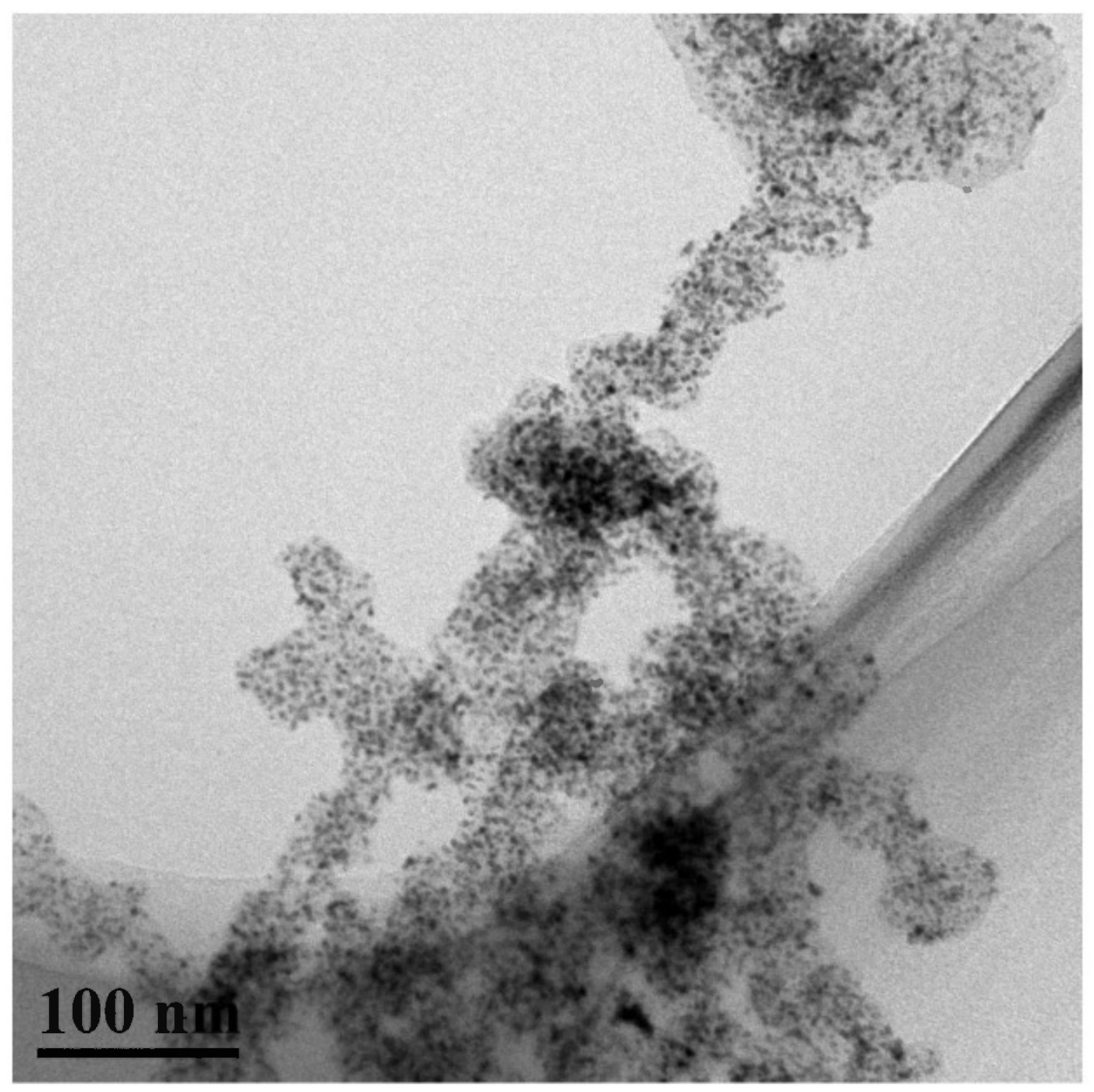
This work illustrates how the presence of a phosphorus-rich surface layer on Pt catalysts enables favorable ORR performance and longevity.
-
The premium version includes another two patent discussions, plus an Excel list with 50-100 commercially relevant recent patent families.
-
Triweekly patent lists for other categories (Excel files are included for premium users)
-
- Lithium metal batteries (excluding Li-S, Li-Air): XLSX
-
- Lithium-ion batteries – electrolytes – liquid: XLSX
-
- Lithium-ion batteries – separators: XLSX
-
- Lithium-sulfur batteries: XLSX
-
- Lithium-air batteries: XLSX
-
- Na-ion batteries: XLSX
-
Prior patent updates
-
2025-03-18
-
2025-02-25
-
2025-02-04
-
2025-01-14
-
2024-12-23
|