-
Lithium-ion batteries – electrolytes – solid & semi-solid
-
N-methyl-N-butylpyrrolidinium ∙ CB9H10, LiCB9H10 and LiCB11H12
(molar ratio 2 : 4 : 4) were heated until a melt was obtained that was subsequently mixed (6 h, temperature not disclosed),
followed by rapid cooling.
Negative electrode formulation: Si / electrolyte described above / Li2S ∙ P2S5 / VGCF
(vapor-grown carbon fibers) = 46 : 18.4 : 27.6 : 8 (by volume).
Positive electrode formulation: NMC111 / Li2S ∙ P2S5 / VGCF (ratio not disclosed).
Solid electrolyte layer formulation: Li2S ∙ P2S5.
Through dry molding in a cylinder, cells were fabricated, which were tested with a 0.2 Nm and a 2 Nm constraint.
A discharge capacity ratio of ≈99% was observed, as compared to ≈91% for comparative cells with negative electrodes based
solely on sulfide electrolyte.
This favorable effect is explained by the higher mechanical flexibility of carborane-based electrolytes
as compared to sulfide electrolytes.
This work illustrates how the novel class of carborane-based solid electrolytes enable operation of
sulfide-based cells at reduced pressure, probably because of a well-rounded combination of mechanical flexibility,
ion conductivity and electrochemical stability near 0 V vs. Li+/Li.
-
The premium version includes another two patent discussions, plus an Excel list with 50-100 commercially relevant recent patent families.
-
Lithium-ion batteries – positive electrode
-
Lithium iron manganese phosphate was prepared by mixing lithium carbonate, ferrous sulfate, manganese carbonate,
monoammonium phosphate, and ascorbic acid in a PTFE hydrothermal kettle with oleylamine.
After stirring to form an emulsion, the mixture was stirred at 150°C for 10 h, followed by centrifugation and washing with ethanol.
The precipitate was dried (80°C, 12 h), followed by a heat treatment (700°C, nitrogen atmosphere, 8 h).
As shown in the TEM (transmission electron microscopy) image below, well-dispersed nanoparticles with a narrow particle size distribution were obtained
(5-15 nm),
coated with carbon, which exhibit favorable electrochemical characteristics according to the voltage diagram as shown in the Figure below
(实施例1: example 1).
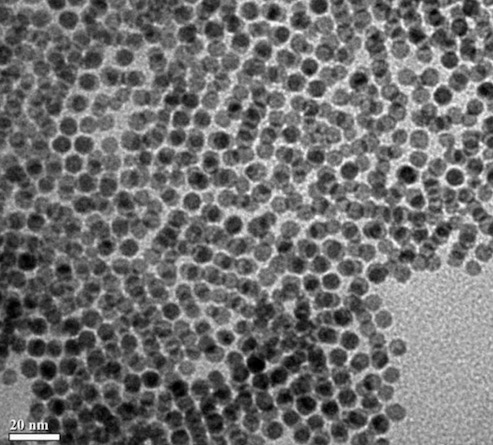
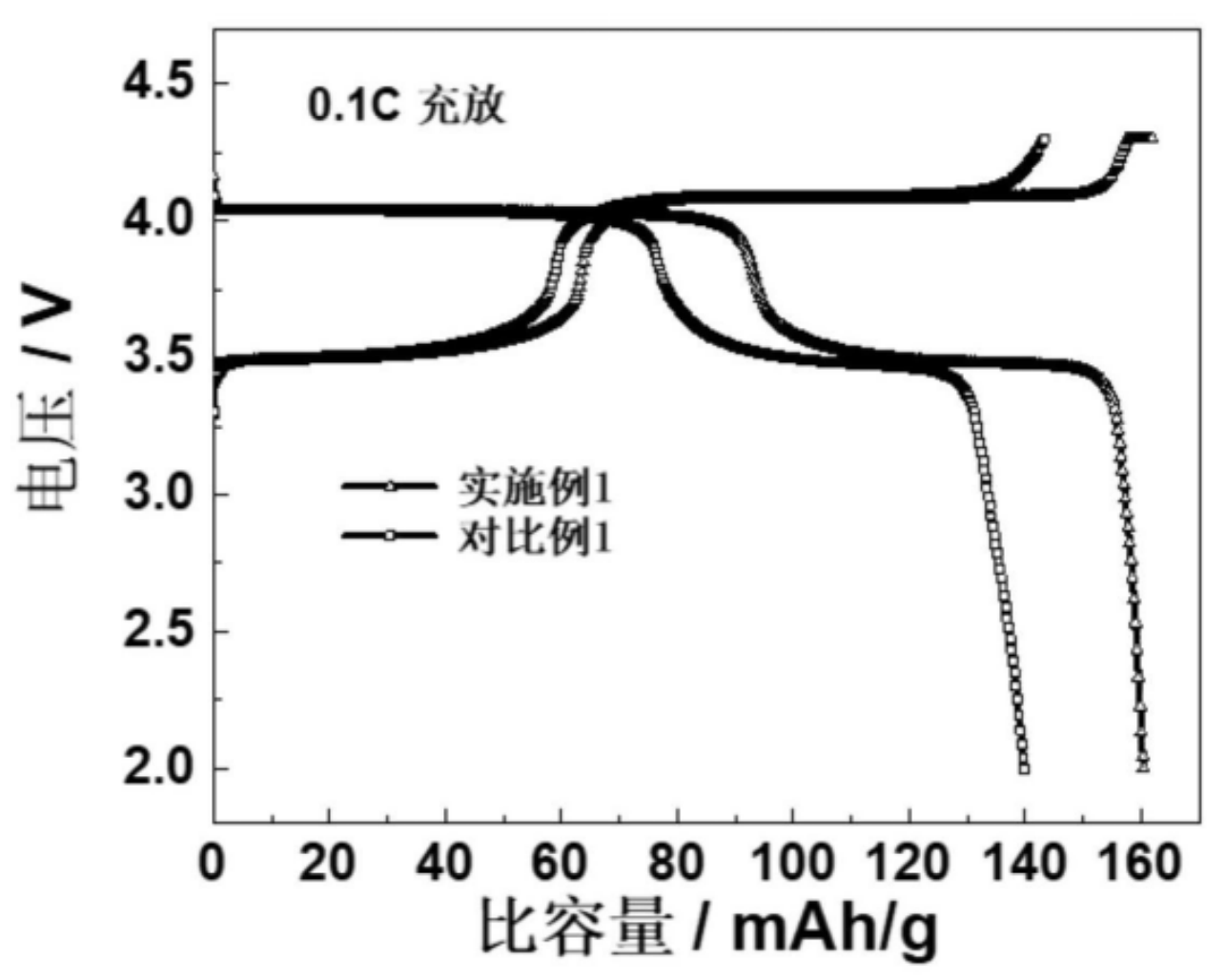
This work illustrates an approach to produce LMFP nanoparticles that likely can be upscaled with attractive raw material and
process costs.
The favorable capacity at 4 V vs. Li+/Li suggests that a Mn / Fe ratio of 7 : 3 or higher might have been used,
although the specific ratio was not disclosed.
-
The premium version includes another two patent discussions, plus an Excel list with 50-100 commercially relevant recent patent families.
-
Lithium-ion batteries – negative electrode (excluding Li metal electrodes)
-
A porous carbon substrate with 2-100 nm apertures was placed
in a CVD (chemical vapor deposition) chamber, purged with nitrogen. Argon gas was introduced before heating to 500°C. Silane gas was
added to form a composite material.
Sodium oxide (0.06 mass%) and magnesium oxide (0.03 mass%) were mixed with the composite material and sintered (600°C, 5 h, box furnace),
followed by CVD coating with carbon (ethylene / argon, 600°C, 10 Torr, 30 min).
In half-cell tests, the material exhibits a discharge capacity of 1,695 mAh/g, a first cycle efficiency of 89.8%, and a capacity retention
after 100 cycles of 92.1% (C-rate not identified).
Although no direct comparative data is shown for a Na- & Mg-free material, this work suggests that the simultaneous presence of sodium oxide
and magnesium oxide between a Si-carbon composite material and its carbon surface coating leads to improved capacity retention.
-
The premium version includes another two patent discussions, plus an Excel list with 50-100 commercially relevant recent patent families.
-
Fuel cells (PEMFC / SOFC / PAFC / AEMFC) – electrochemically active materials
-
Catalytically active Pt0.40Cu0.20Mo0.40 nanoparticles as shown in the SEM image below were prepared
by ultrasonicating platinum(II) acetylacetonate, copper(II) acetylacetonate, oleic acid, oleylamine in diphenyl ether (30 min).
The solution was heated (220°C, 30 min), followed by the addition of molybdenum hexacarbonyl, followed by heating for another 30 min.
The platinum-based alloy was cleaned with ethanol and cyclohexane, then suspended in 30 ml of cyclohexane.
Ketjen black 300JD in cyclohexane was combined with the platinum alloy solution to achieve a 31.6 mass%
loading on the carbon support, followed by ultrasonication. The resulting powder was mixed with acetic acid, ultrasonicated,
centrifuged, and dispersed in an acetone-ethanol mixture, ultrasonicated again, and vacuum dried.
Upon preparing a Nafion-based catalytic membrane, oxygen reduction reaction (ORR) characteristics as shown in the Figure below
were obtained (measurement of geometric current density in the context of an accelerated durability test,
0.05-0.9 V at sweep rate of 200 mV/s at pH 0.1).
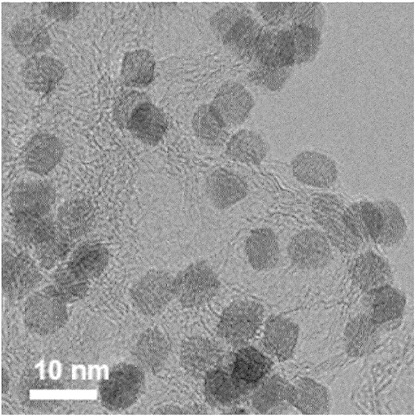
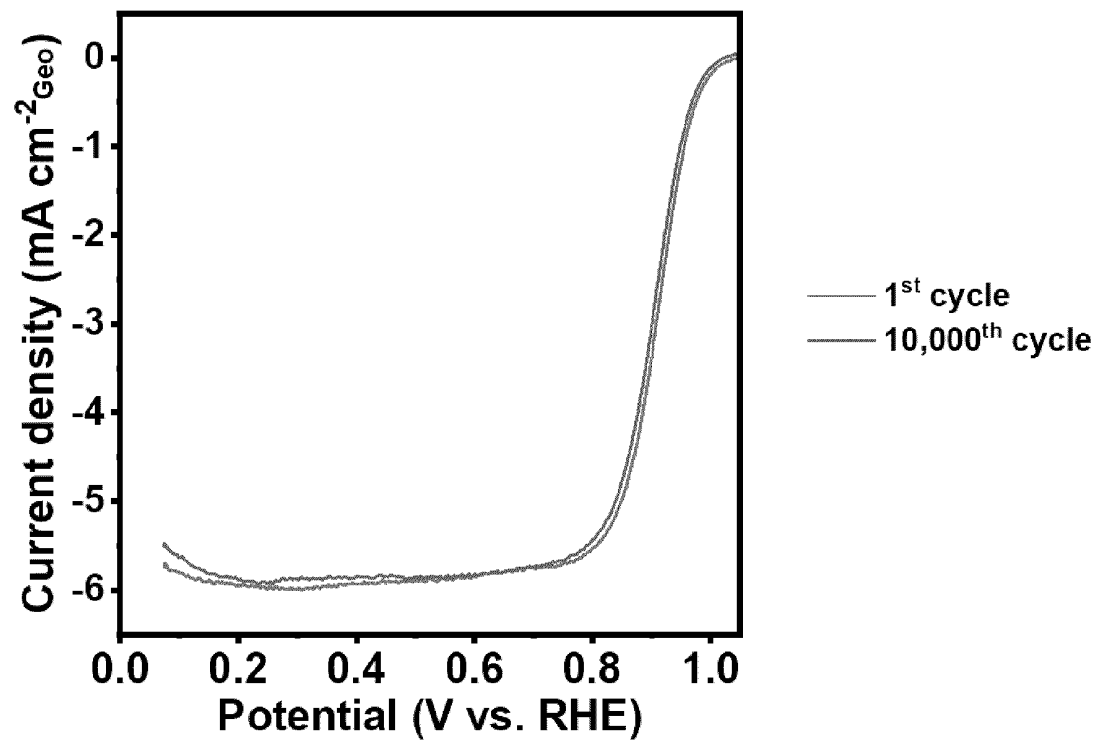
This work suggest that the combination of Pt / Cu / Mo in an alloy results in resilient catalytic nanoparticles.
-
The premium version includes another two patent discussions, plus an Excel list with 50-100 commercially relevant recent patent families.
-
Triweekly patent lists for other categories (Excel files are included for premium users)
-
- Lithium metal containing batteries (excluding Li-S, Li-Air): XLSX
-
- Lithium-ion batteries – electrolytes – liquid: XLSX
-
- Lithium-ion batteries – separators: XLSX
-
- Lithium-sulfur batteries: XLSX
-
- Metal-air batteries: XLSX
-
- Na-ion batteries: XLSX
-
Prior patent updates
-
2024-02-13
-
2024-01-23
-
2024-01-02
-
2023-12-12
-
2023-11-21
|