-
Deep Dive – Recent Patents & Innovations in Lithium Manganese Iron Phosphate (LMFP) Cathode Materials
11 pages, addendum with patent summaries: 41 pages, version: 2025-01-03
-
Introduction
LMFP (lithium manganese iron phosphate) has emerged as a promising cathode active material for lithium-ion batteries, combining advantages of both LFP (lithium iron phosphate, low raw material costs, favorable inherent safety profile) and manganese-rich chemistries (higher voltage than LFP, resulting in 15-20% higher energy density). This deep dive covers key LMFP-related product development decisions through an analysis of newly published patents (since 2023) and publicly disclosed information from key commercial players.
LMFP product & process definition efforts involve the following aspects (Figure B-1):
-
Optimization of manganese / iron ratio to balance performance and stability (Figure B-2)
-
Various doping strategies using elements like titanium, niobium, and boron (Figure B-3)
-
Surface modifications (Figure B-4) and core-shell / gradient architectures (Figure B-5)
-
Particle size distribution and morphology control (Figure B-6)
-
Choice of manufacturing process steps (Figure B-7)
-
Positive electrodes based on active material blends (Figure B-8)
-
The sections below are included in the full version.
-
Perspectives for LMFP – Academic Viewpoint
-
LMP (Lithium Manganese Phosphate)
-
LMFP (Lithium Manganese Iron Phosphate)
-
Sustainability
-
State of LMFP Commercialization
-
Table B-1: Summary of Key LMFP Commercialization Efforts (full version: 15 entries)
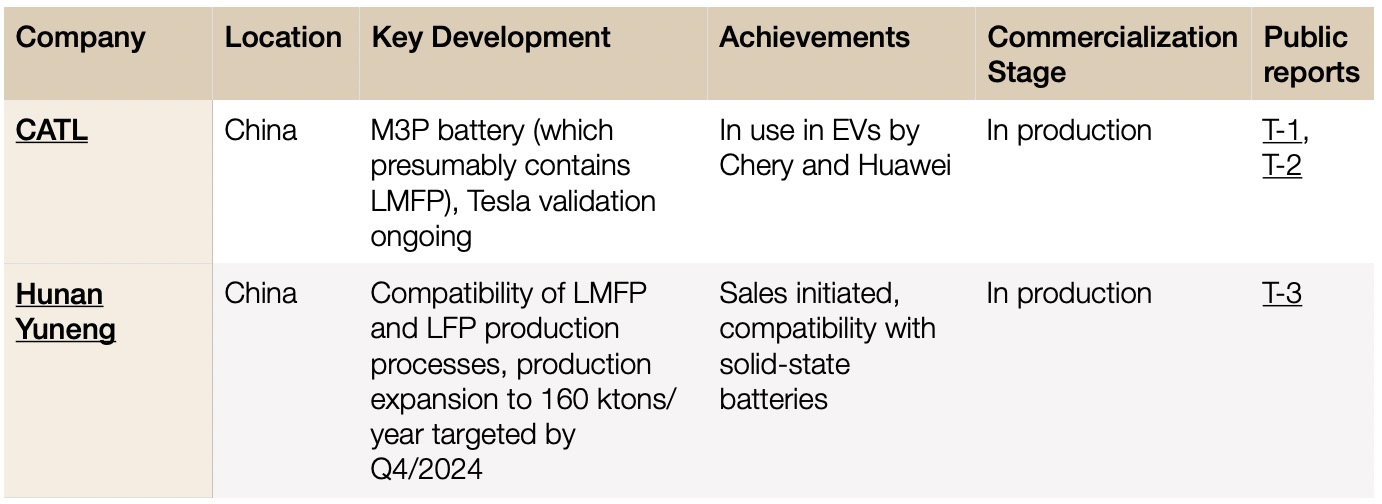
-
Product Development Decisions
-
Figure B-1: Technology Decision Tree – Product Development Decisions (Overview)
-
Figure B-2: Technology Decision Tree – Choice of Mn / Fe Molar Ratio
As shown in Figure B-2, key commercial players have explored Mn / Fe molar ratios ranging from 99% Mn (CATL) to 10% Mn (Mitra Future Technologies). Other active materials exhibit Mn / Fe ratios in between (most common Mn ratios: 60-80%, AESC, BYD, 2nd patent, CATL: 2nd, 3rd, 4th patents, Dynanonic, Dynanonic / Qujing Defang, Easpring, Eve Energy, Guoxuan / Gotion, 2nd patent, HCM, Integrals Power, Livium / VSPC, Panasonic, Ronbay, 2nd patent, Tianjin Rongbai Sikelande – Rongbai subsidiary, 2nd, 3rd patents, SVOLT, 2nd patent, Taiheiyo Cement, Wanxiang 123).
Links above point to patent summaries in addendum.
-
Figure B-3: Technology Decision Tree – Doping
-
Figure B-4: Technology Decision Tree – Core-shell / Gradient Architectures
-
Figure B-5: Technology Decision Tree – Surface Modifications
-
Figure B-6: Technology Decision Tree – Particle Morphology & Size Control
-
Figure B-7: Technology Decision Tree – Synthesis Methods (Core)
-
Figure B-8: Technology Decision Tree – Blends
-
Outlook
-
Addendum (with links to sections above)
-
Patent Summaries Added During Preparation of This Deep Dive
-
Triweekly Patent Updates – Lithium-ion Batteries – Positive Electrode – LMFP – 2023-02-08 until 2024-12-23
|